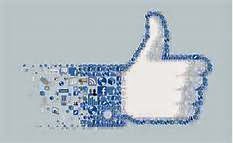.jpg)
Hi - At a glance;
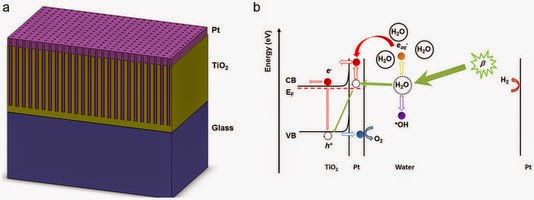
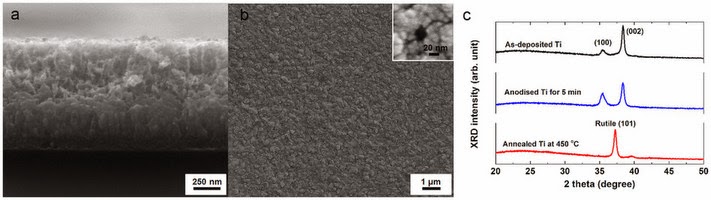
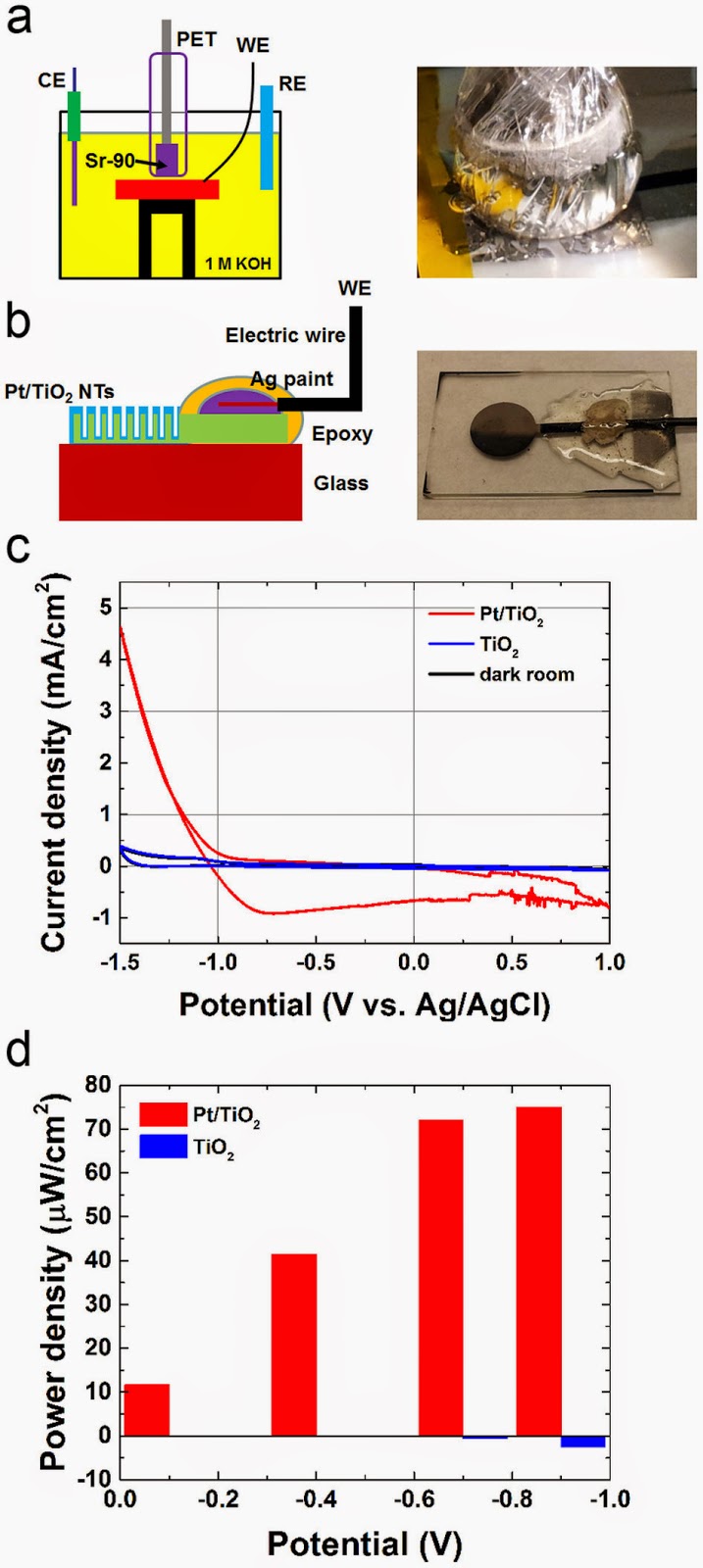.jpg)
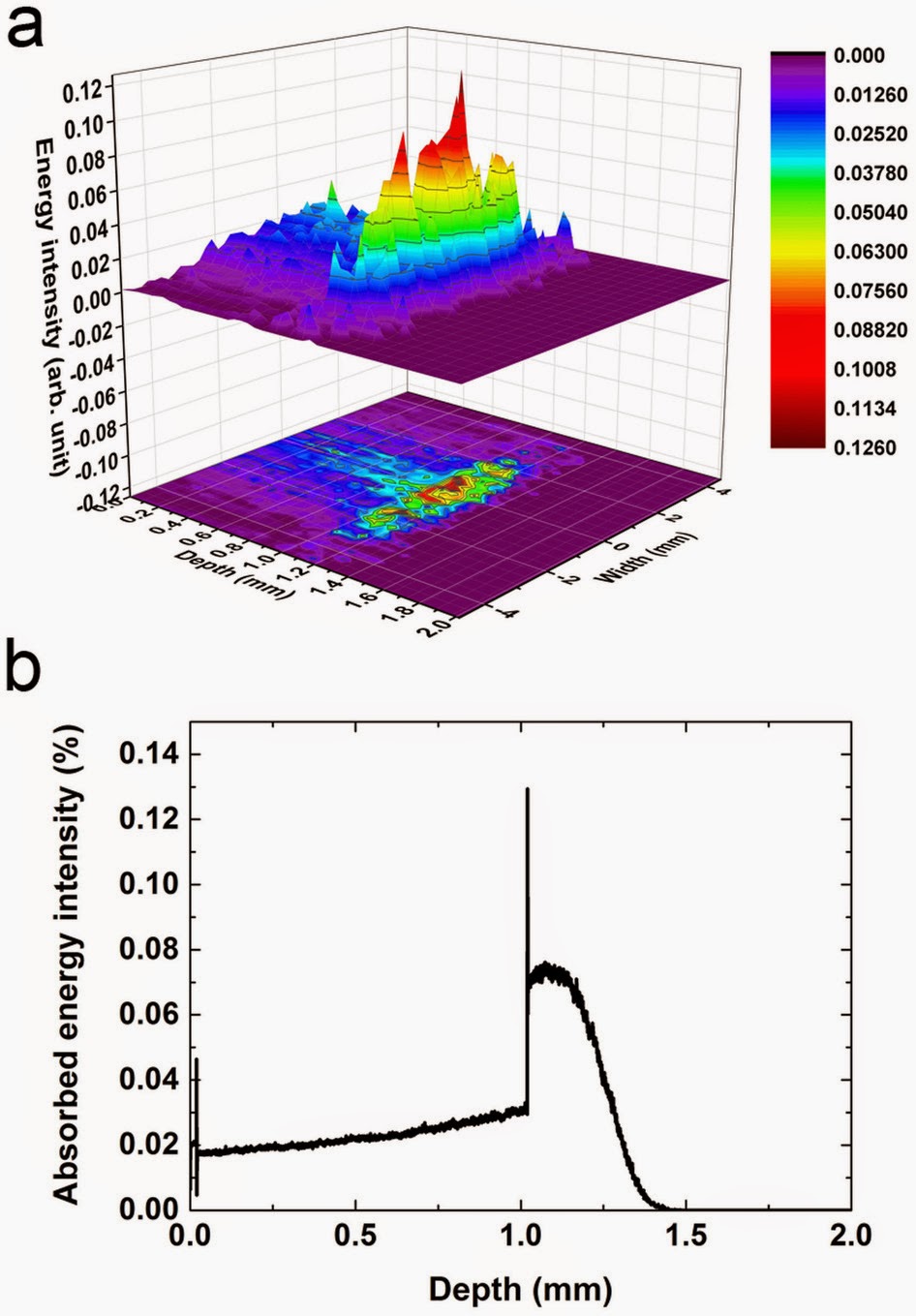.jpg)
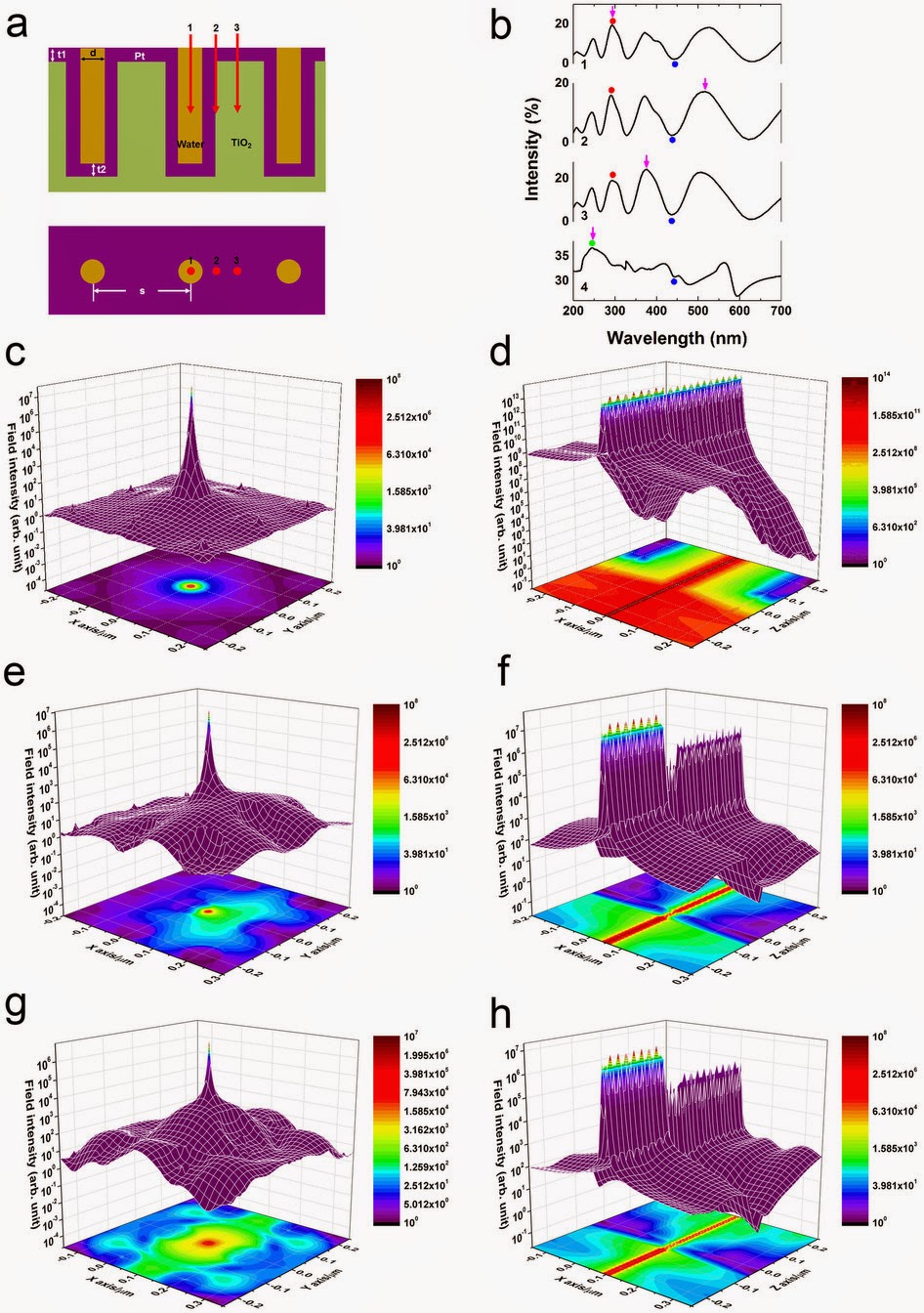.jpg)
Hi - Introduction:
Hi - Design and operating principle of Pt/nanoporous
TiO2 radiolytic cell:
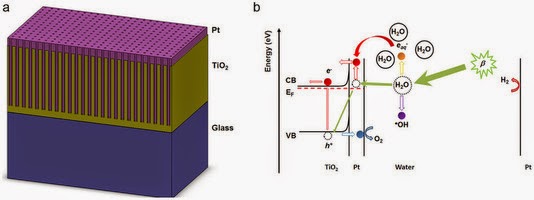.jpg)
Hi - Structural properties of radiolytic electrode:
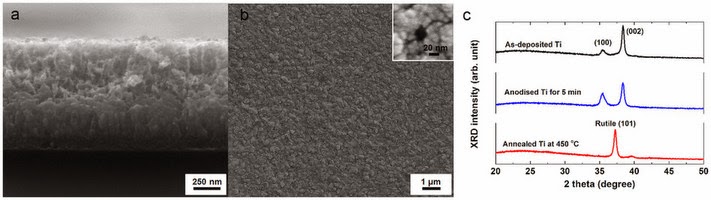
Hi - Electrical properties of radiolytic cell:

TiO2 electrode:
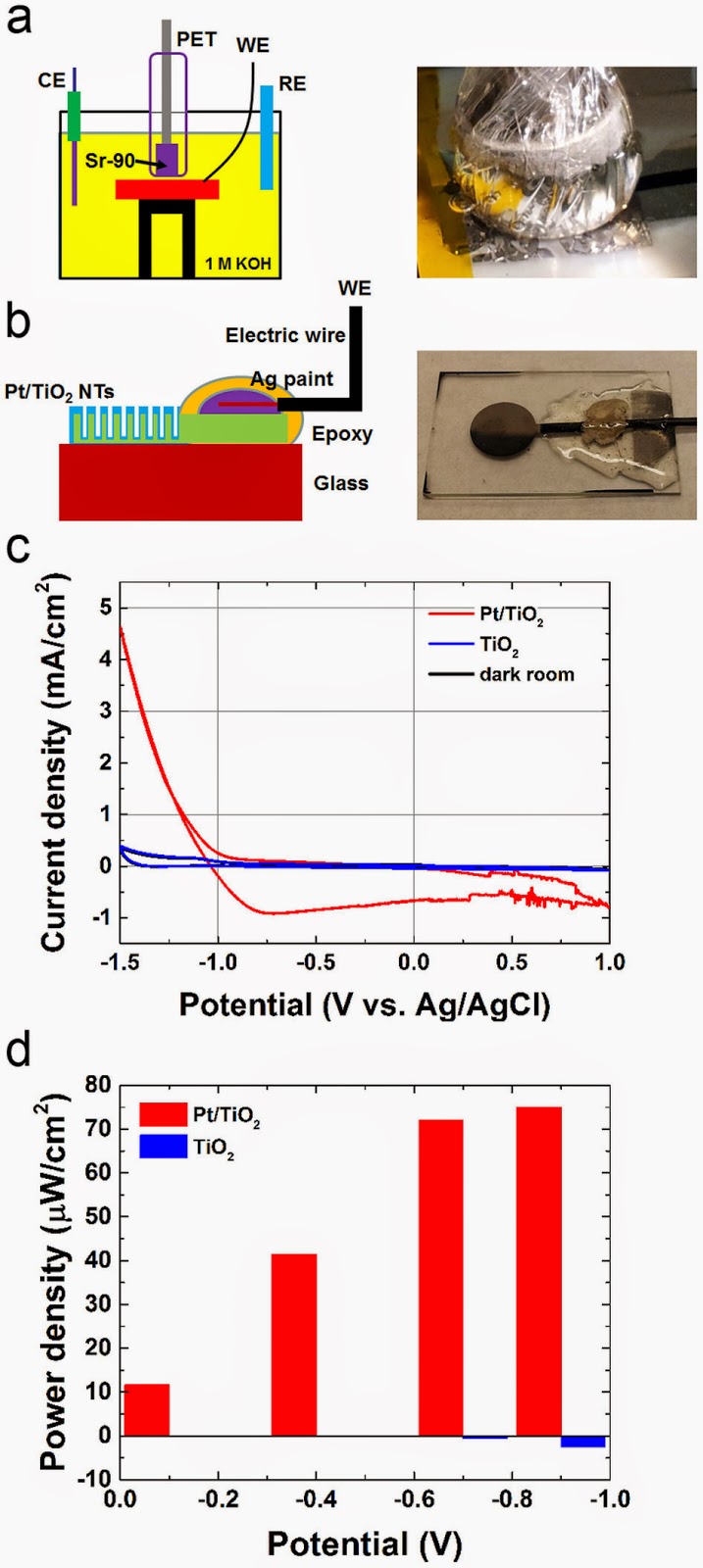.jpg)
Hi - Energy absorption of electron beam in the radiolytic
cell using numerical simulation:
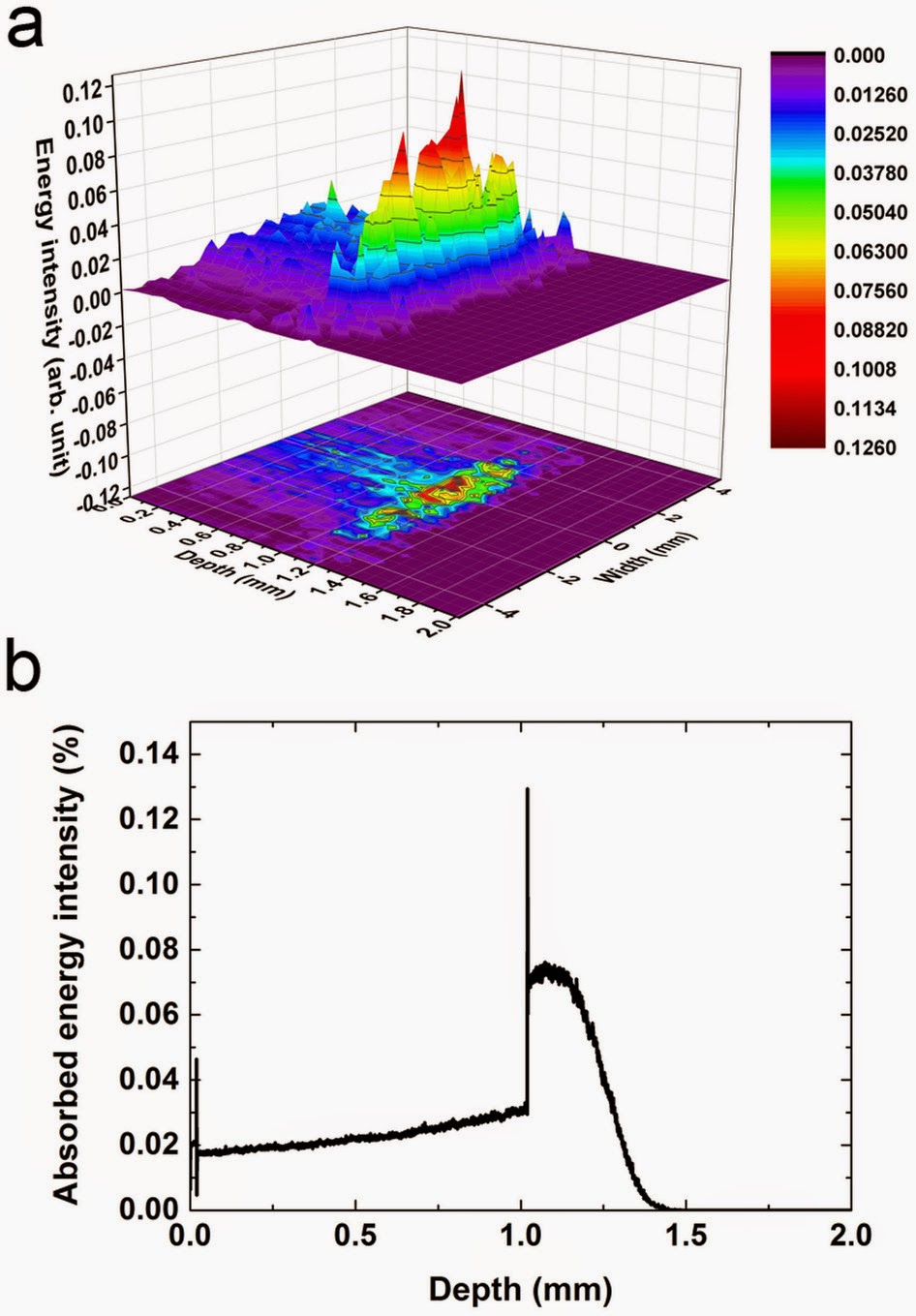.jpg)
Hi - Generation and behaviour of free radicals under beta radiation:

FDTD simulations of Pt/nanoporous TiO2 on glass under an e-beam irradiation of 546 keV and optical measurement

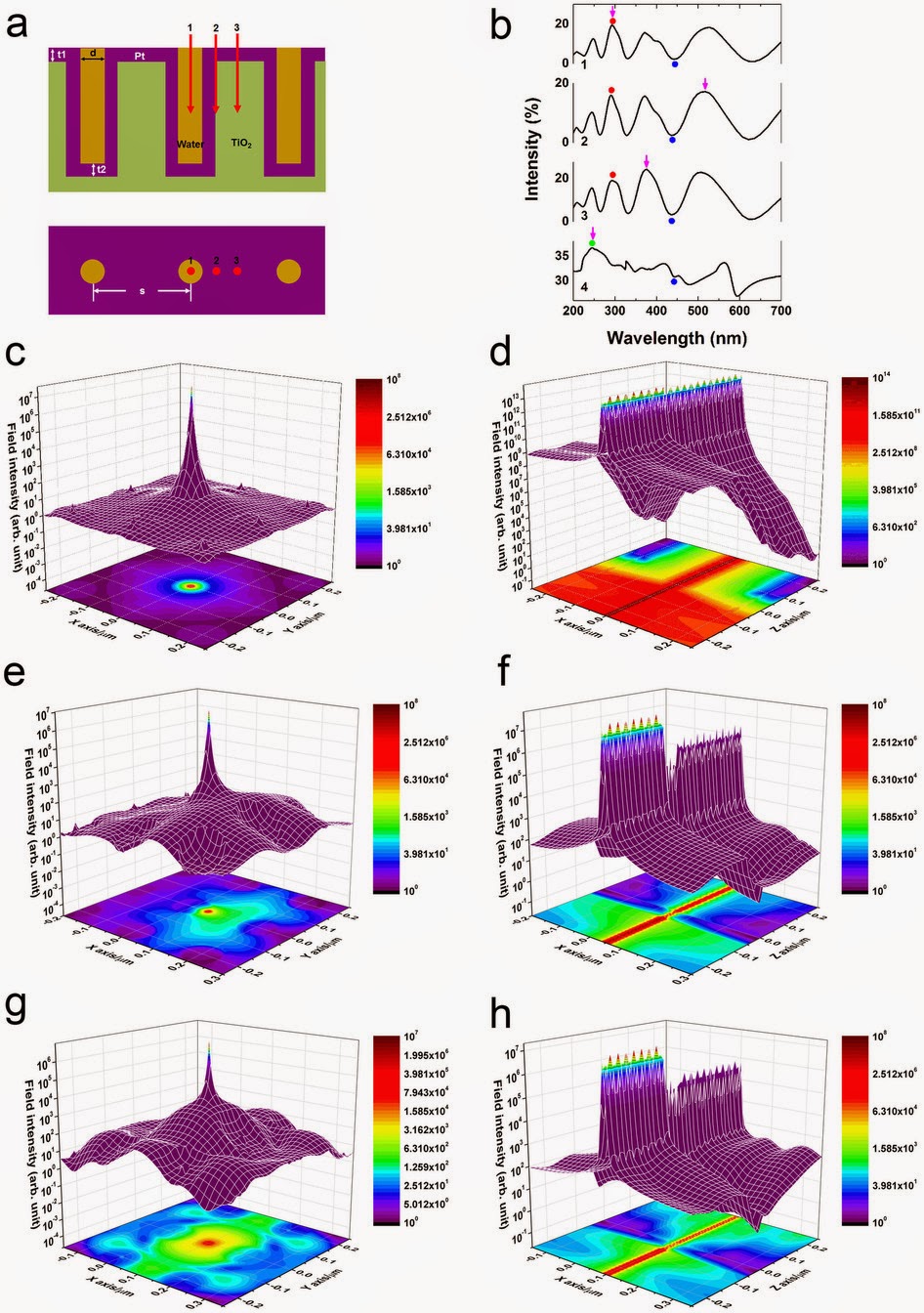.jpg)
(a), Cross-sectional view and top view for different e-beam positions marked as 1–3. In this simulation, d, s, t1, and t2 are 10 nm, 200 nm, 50 nm, and 45 nm, respectively. (b), Emission spectra of the Pt/nanoporous TiO2 surfaces at positions 1–3. Arrows indicate the highest peak positions. Mark 4 shows the reflection of Pt/nanoporous TiO2 using an UV-VIS spectrometer. The same coloured dots indicate corresponding peaks of each spectrum. (c–d), Top and cross-sectional views of the near-field intensity distributions on Pt/nanoporous TiO2 at the top level of Pt nanohole at mark 1 for 290 nm. (e–f), Top and cross-sectional views of the near-field intensity distributions on Pt/nanoporous TiO2 at the top level of Pt nanohole at mark 2 for 287 nm. (g–h), Top and cross-sectional views of the near-field intensity distributions on Pt/nanoporous TiO2 at the top level of Pt nanohole at mark 3 for 377 nm. The field intensity is shown on a log scale, [E]2/[E0]2, where E and E0 are the generated and minimum electric field intensities, respectively.
To simulate the near-field electric intensities and emission spectra at the different positions, the induced electric field distributions were calculated by [E]2/[E0]2, where E and E0 are the generated and minimum electric field intensities, respectively, emitted normal and parallel to the Pt surface in the wavelength range of 200 to 700 nm. We investigated the near-field intensity distributions on Pt/nanoporous TiO2 at the different positions under focused electron irradiation using FDTD simulations. As shown in Fig. 5b, the highest emitted spectral peaks were calculated to occur at wavelengths of 293 nm, 517 nm, and 376 nm when the electron irradiation is focused near positions 1, 2, and 3, respectively. These results indicate that the strongest surface plasmon energy (4.23 eV) and field intensity are generated when the nanohole is irradiated. Such strong surface plasmons have been observed in Pt nanostructures at similar energy levels due to an interband transition of the particles. The diffraction pattern is also shown for position 3 on periodic Pt/nanoporous TiO2. To confirm the surface plasmons, we measured the reflectance of Pt/nanoporous TiO2 structures using an UV-VIS spectrometer. As shown in Fig. 5b, marks 1–3 are the calculated emission spectra of Pt/nanoporous TiO2 in water, while mark 4 is the measured reflectance of Pt/nanoporous TiO2 in air. The reflection peaks (colour dots) of mark 4 were found at the similar positions with respect to the simulated emission peaks shown at marks 1–3. The strongest reflection peak (purple arrow) of Pt/nanoporous TiO2 is shown at 245 nm (5.06 eV) by plasmon resonant coupling in air/Pt nanoholes. To understand the surface plasmon phenomena between two materials, we have to solve Maxwell's equation for the electromagnetic wave at the interface between two materials, which has relative dielectric functions ε1 ( = ε′1 + iε″1 = (n12 − κ12) + i(2n1κ1)) and ε2 ( = ε′2 + iε″2 = (n22 − κ22) + i(2n2κ2)) with the appropriated continuity relationship. Real and imaginary parts of the dielectric function are presented. The nj and κj are refractive index and extinction coefficient, respectively, and j is 1 or 2. By boundary conditions, the dispersion relation for a wave propagating on the surface is

Hi - Discussion:
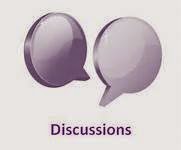.jpg)